On the 9th June 2021, the MAREWIND consortium gathered to discuss the project status and progress since its official launch in December 2020. The MAREWIND consortium partners met online due to the current COVID-19 restrictions across Europe.
Progress
During the 1-day online session, all partners presented the work done for each of the work packages and their main achievements. Members of the consortium discussed the next significant milestones and deliverables that are to come. In addition, the consortium also detailly presented the planned actions in short term. By the end of the meeting, the project coordinator has concluded that the project and work packages are going as foreseen with no delays.
Next steps
The consortium is preparing its first newsletter, so stay tuned for more detailed project progress. You can subscribe for the latest MAREWIND news and events, here.
The next consortium meeting is scheduled in November 2021 when first year of the project would be achieved. For now the meeting will be held online again.
Have you ever wondered why wind energy is so important? Nowadays, it is the most efficient technology to produce clean power for several industries and electricity for the people in a safe and environmentally sustainable way.
These benefits motivate European governments to look for investment and further development in the wind energy sector as key Renewable Energy Source (RES). In this context, how is wind energy produced?
What is wind energy?
Wind energy/power is the energy that comes from a natural or renewable resource. It is actually a by-product of the sun. The wind energy is clean, use less water and does not produce any greenhouse gas emissions or air pollutions. The wind energy is a green alternative to the energy produced by burning fossil fuels.
Wind energy is actually a by-product of the sun. The sun’s uneven heating of the atmosphere, the earth’s irregular surfaces (mountains and valleys), and the planet’s revolution around the sun all combine to create wind. Since wind is in plentiful supply, it’s sustainable resource for as long as the sun’s rays heat the planet.
What is wind turbine ?
A wind turbine captures the kinetic energy from the wind and converts it into mechanical power or simply electricity. It produces energy in a safe and environmentally sustainable way.
There are 2 types of wind turbines:
- Horizontal-axis wind turbines are the most commonly used one due to their strength and efficiency. They commonly have 3 blades like airplane propellers. Nearly all of the wind turbines currently in use are horizontal-axis turbines.
- Vertical-axis turbines are less used today as they do not perform as well as the horizontal-axis turbines. They have blades that are attached to the top and the bottom of a vertical rotor.
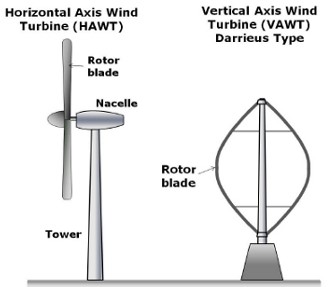
Figure 1: Types of wind turbines.
How is the wind turbine work?
When wind flows across the blade, the air pressure on one side of the blade decreases. The difference in air pressure across the two sides of the blade creates both lift and drag. As the force of the lift is stronger than the drag, it makes the rotor to spin. Then, the rotor connects to the generator and speed up the rotation. This creates electricity.
Figure 2: How does a wind turbine work?
Credits: ACCIONA, MAREWIND’s partner
What is wind farms?
A wind farm is a group of wind turbines that produce large amounts of electricity. Its aim is to deliver power to the electrical grid. Wind farms can be placed on land (onshore) or fixed at the sea (offshore). Offshore wind farms can even have floating turbines in deep waters.
Figure 3: Types of wind farms. Credits: TUV SUD
Infographic
Offshore wind turbines are often exposed to a variety of harsh conditions such as abrasion, bio-fouling, corrosion. These conditions seriously damage the components of the wind turbines. Moreover, due to the constant exposure of the marine moisture, splash and ice formations and based on the location of the windmill plan, some of the turbines have more severe damages. Overall, these conditions significantly decrease the lifespan of the wind turbines and limit the cost per MW.
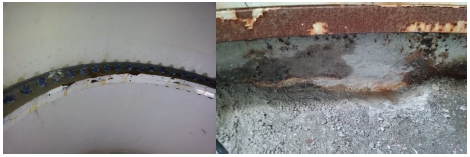
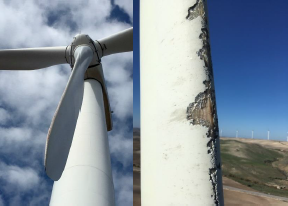